Introduction: Normal Cell Metabolism
Cellular respiration describes the series of steps that cells use to break down sugar and other chemicals to get the energy we need to function. Energy is stored in the bonds of glucose (like a stretched rubber band), and when glucose is broken down, much of that energy is released. Some of it is captured in a form that can be used to do work in cells - a molecule called adenosine triphosphate or ATP. The energy that is not captured in ATP is usually given off as heat (one of the things that helps us maintain our normal body temperature).
The process of cellular respiration is similar to a car using gasoline as fuel. As gasoline is the fuel for a car, glucose is the fuel for a cell. A car burns gasoline and uses the energy released for movement. Similarly, a cell ‘burns’ glucose to capture the energy and create ATP. ATP is the primary form of energy that cells use to function.
The first step of respiration is called glycolysis. In a series of steps, glycolysis breaks glucose into two smaller molecules - a chemical called pyruvate. A small amount of ATP is also formed during this process. The process can be likened to a waterslide. A person has more energy at the top and loses it as they slide down.
Most healthy cells continue the breakdown in a second process, called the Kreb's cycle. The Kreb's cycle allows cells to “burn” the pyruvates made in glycolysis to get more ATP.
The last step in the breakdown of glucose is called oxidative phosphorylation (Ox-Phos). It takes place in specialized cell structures called mitochondria. This process produces a large amount of ATP. Importantly, cells need oxygen to complete oxidative phosphorylation. If a cell completes only glycolysis,only 2 molecules of ATP are made per glucose. However, if the cell completes the entire respiration process (glycolysis - Kreb's - oxidative phosphorylation), about 36 molecules of ATP are created, giving it much more energy to use. Further information on the topics on this page can also be found in most introductory Biology textbooks, we recommend Campbell Biology, 11th edition.1
Learn more about mitochondria and energy production.
Topics on this Page:
- What Cancer Cells Do Differently
- Hypoxia and the Tumor Environment
- Genetic Changes and Cancer Cell Metabolism
- Metabolism in Tumor Detection and Treatment
What Cancer Cells Do Differently
Unlike healthy cells that "burn" the entire molecule of sugar to capture a large amount of energy as ATP, cancer cells are wasteful. Cancer cells only partially break down sugar molecules. They overuse the first step of respiration, glycolysis. They frequently do not complete the second step, oxidative phosphorylation. This results in only 2 molecules of ATP per each glucose molecule instead of the 36 or so ATPs healthy cells gain. As a result, cancer cells need to use a lot more sugar molecules to get enough energy to survive. 2
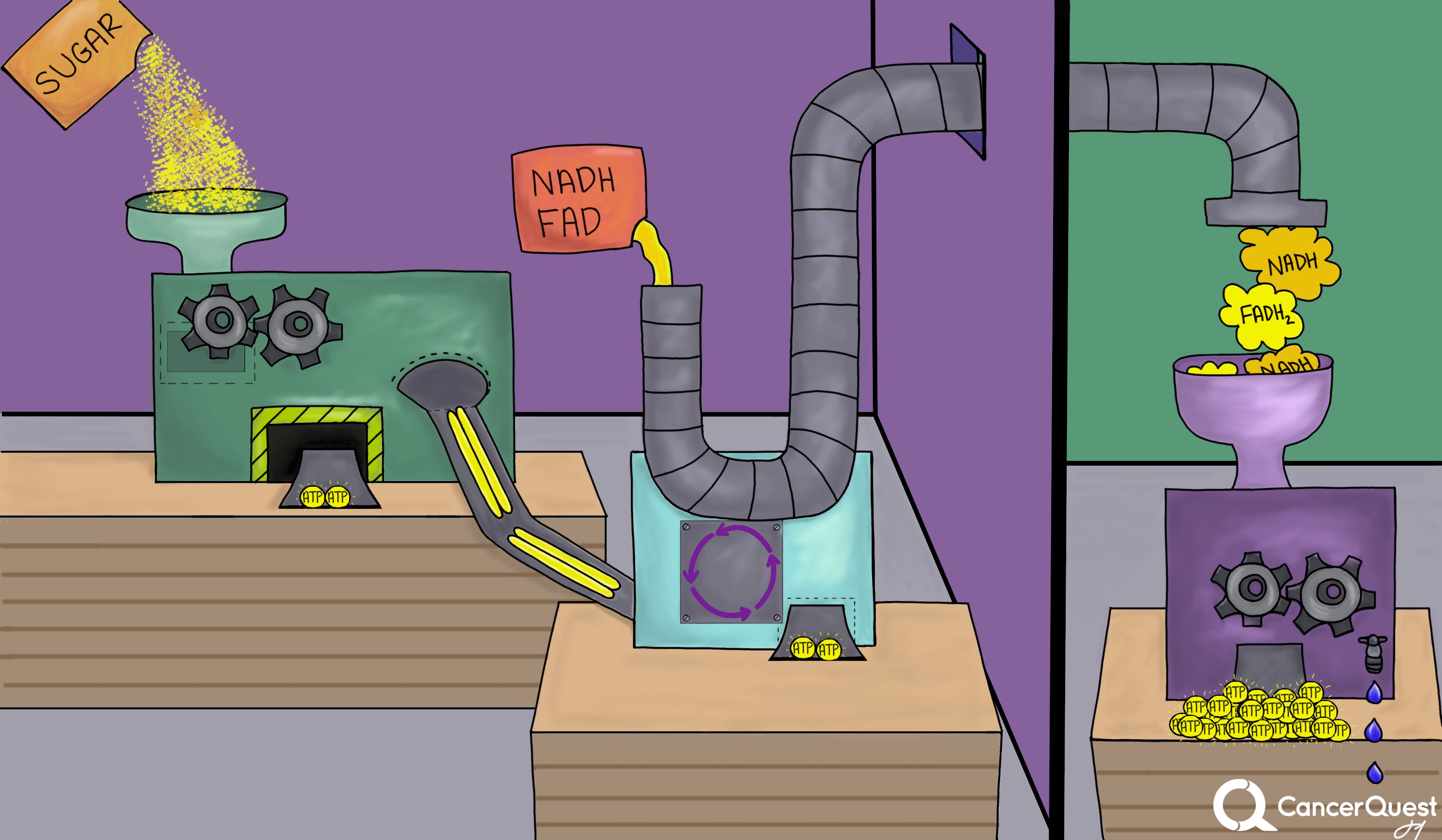
Production of usable energy - ATP (yellow balls) - in normal cells. The process has three main steps. The first step is glycolysis and it produces only a small amount of ATP. The majority of the ATP is made in the next two steps (the Kreb's cycle and the electron transport chain or ETC).
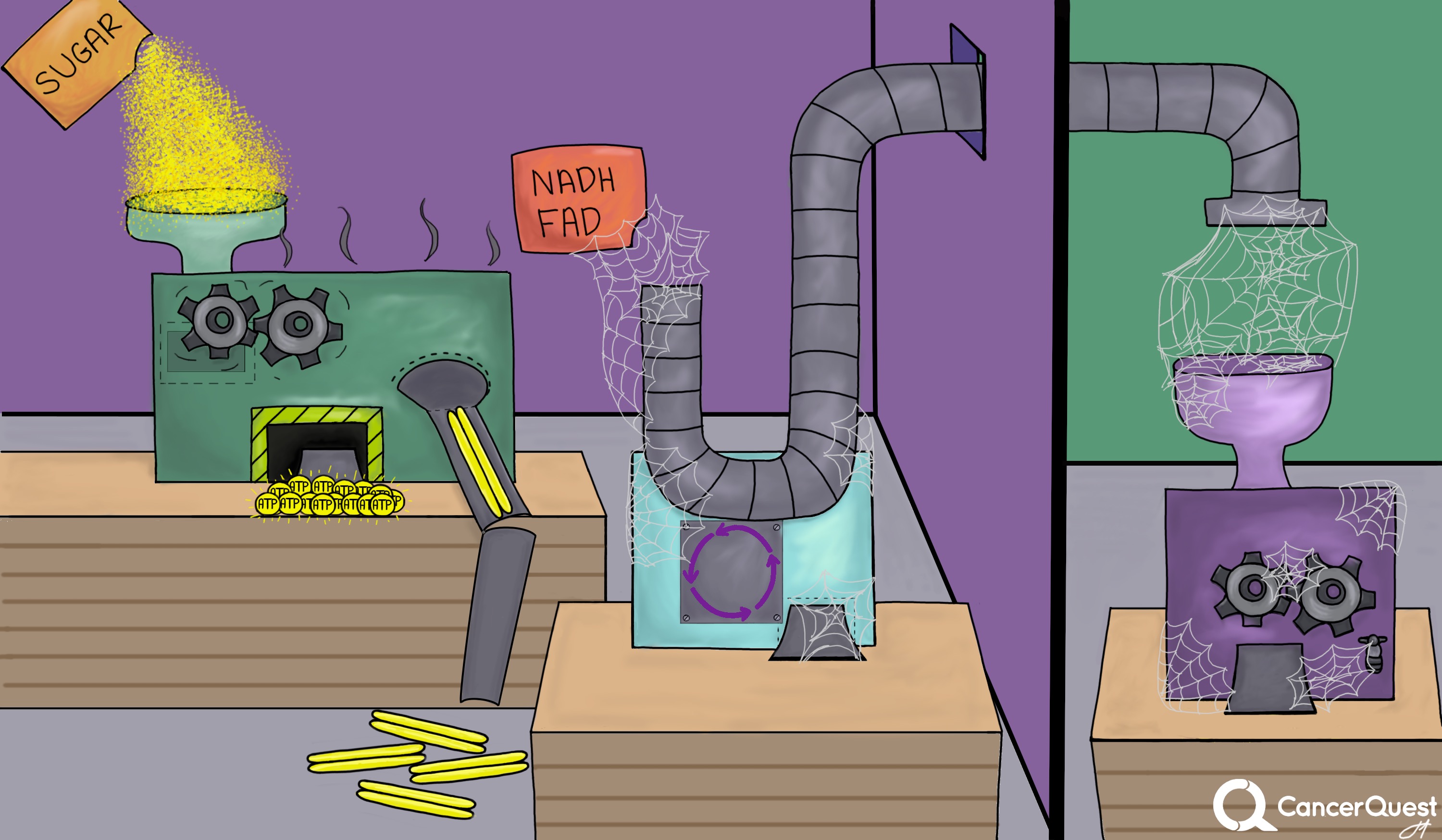
Otto Warburg, a German scientist, was the first to describe this unusual behavior of cancer cells. He won the Nobel Prize in 1931 for his work. He noticed that cancer cells only complete glycolysis (and NOT Ox-Phos), even when oxygen is present (a process called aerobic glycolysis). The presence of oxygen should allow them to complete the entire process of respiration. An abnormal dependence on glycolysis as the sole source of ATP creation, even in the presence of oxygen is seen in many cancer cells and is commonly called the 'Warburg effect'. 3
Some cancer cells may not be able to complete the entire respiration process due to defects caused by changes in their DNA (mutations), but that is not the whole story. Using only glycolysis may provide cancer cells with some advantages. The products of glycolysis can be used to build products that help cancer cells to survive and grow.
Research has also suggested that using aerobic glycolysis may help cancer cells avoid being recognized and killed by cells of the immune system. 2 Changes in the metabolic environment may block immune cells from finding cancer cells and even attract cells that may help tumor cells to grow. The unusual metabolic changes seen in cancer cells may also activate oncogenes that allow the cancer cells to avoid death.
Hypoxia and the Tumor Environment
The environment within a tumor is stressful for the normal cells living there. The blood vessels (vasculature) in a tumor are not formed properly and are often twisted and abnormal (convoluted) looking. The defective structure leads to a poor ability to deliver oxygen and results the development acidic conditions. Another result of the abnormal vessel distribution is that some parts of the tumor are far from blood vessels and do not receive enough nutrients and oxygen. 4 As tumors grow in size, they can outgrow their blood supply. This results in the area inside the tumor becoming very low in oxygen (hypoxic). Cells that only use glycolysis are not dependent on oxygen for survival. This may benefit cancer cells that are in environments low in oxygen.
In response to hypoxic conditions (aka. hypoxia), a protein called hypoxia induced factor 1-alpha (HIF1-α) is activated. The HIF1-α protein increases the rate of glycolysis and decreases the conversion of glucose to the products seen in normal cells. 5 Hypoxia and HIF1-α activation cause problems. Low oxygen levels help to promote cell movement and cancer spread (metastasis) by causing the production of TWIST, a protein that plays an essential role in metastasis. TWIST activation results in cancer cells loosening their grip on their surroundings, allowing them to move and invade nearby tissues. The process by which epithelial cells change into a type of cell that is able to move more easily is known as the epithelial-mesenchymal transition (EMT). Along with the ability to move, the EMT gives the cells additional ‘primitive’ capabilities that help protect the cancer cells and enhance the spread of cancer. 6
Genetic Changes and Cancer Cell Metabolism
Many DNA changes (mutations) occur in cancer cells that are not present in healthy cells. Some of these changes can lead to increased glycolysis. AKT, an oncogene involved in cell metabolism and survival, can be activated in response to hypoxia and HIF1-α. This can lead to increased survival of cancer cells. 5 Other oncogenes, RAS and MYC, are often activated in cancer cells. Their proteins both contribute to the aerobic glycolysis seen in cancer cells. 7
In cancer cells, tumors suppressors that stop cancer cell growth and lead to cell death are often inactivated. The loss of the tumor suppressor p53 can trigger the Warburg effect and cells becoming "addicted" to glycolysis. 4
Aerobic glycolysis is also linked to the production/activity of another protein, the vascular endothelial derived growth factor (VEGF). VEGF causes blood vessel formation (angiogenesis). Tumors need to create new blood vessels to retain a nutrient supply as they grow. The abnormal metabolism seen in cancer cells may drive the creation of new blood vessels. 8
Researchers recently discovered another way that cancer cells produce the products they need to survive. Mitochondria in cells use lactate to grow and fuel reactions. Multiple experiments were done on individual mitochondria in cancer cells, and the results confirmed that lactate is getting into the mitochondria and being used to create nutrients for the cancer cell.9
Metabolism in Tumor Detection and Treatment
As described above, cancer cells often rely mainly on glycolysis to produce ATP. This is a very inefficient way to obtain ATP. Cancer cells must therefore use a lot more fuel (glucose) to generate enough ATP to survive. Positron emission tomography (PET) is a detection method that takes advantage of this situation to detect cancer. In PET imaging, patients are injected with a chemical, fluorodeoxyglucose (FDG), that is a slightly changed form of the sugar glucose. The presence of FDG in the body can be detected by a PET machine.
Unlike other imaging methods used to detect cancer, like CT and MRI scans, PET imaging is detecting the activity of the cells, not just their location. PET can be used to stage tumors, follow responses to treatment, predict aggressiveness of tumors, and help to predict patient outcomes. 10
A PET scan with a bright spot showing rapid FDG uptake by a brain tumor.
Cancer cell metabolism also provides clues to possible targets of treatment. Dichloroacetate (DCA) is a chemical that is being tested for its ability to reactivate Ox-Phos in tumor cells and suppress their growth. 11 Clinical trials are also examining the use of drugs that block glycolysis
Cancer Cell Metabolism and the Spread of Cancer (Metastasis)
Why and how cancer cells invade tissues and spread to distant areas of the body is a major unresolved question in cancer biology and is of great importance to cancer patients. At least part of the answer lies in metabolic changes in migrating cells.
Work by Adam Marcus and colleagues on the migration of cancer cells has shown that the cells leaving a model tumor can be divided into two main types: 'leaders' and 'followers'. The leader cells are the ones at the front of the pack. They seem to actively recruit and guide follower cells. Below is a video showing a leader cell leaving a model tumor (this is growing in a laboratory dish, not in a person). When no followers come along, the leader goes back and gets one. Then they migrate away from the 'tumor'.
Interestingly, results published in 2020 by this group shows that leader cells rely on different metabolic pathways than follower cells!! The leaders use the energy production potential of Kreb's cycle and other systems in mitochondria but followers rely much more on glycolysis. This change in energy production ends up changing where mitochondria are found in the cells and may change the way they are able to move. The results also suggest possible ways to target migrating cancer cells.12
- 1Urry, L. A., Cain, M. L., Wasserman, S. A., Minorsky, P. V., & Reece, J. B. (2017). Campbell Biology (11th ed.). Pearson.
- 2 a b Kroemer G, Pouyssegur J. Tumor cell metabolism: cancer's Achilles' heel. Cancer Cell. 2008 Jun;13(6):472-82. [PUBMED]
- 3Warburg, O. On the origin of cancer cells. Science. 1956 Feb 24;123(3191):309-14. [PUBMED]
- 4 a b Denko NC. Hypoxia, HIF1 and glucose metabolism in the solid tumour. Nat Rev Cancer. 2008 Sep;8(9):705-13. [PUBMED]
- 5 a b Kaelin WG Jr, Thompson CB. Q&A: Cancer: clues from cell metabolism. Nature. 2010 Jun 3;465(7298):562-4. [PUBMED]
- 6Wu KJ, Yang MH. Epithelial-mesenchymal transition and cancer stemness: the Twist1-Bmi1 connection. Biosci Rep. 2011 Dec;31(6):449-55. [PUBMED]
- 7Singleterry J, Sreedhar A, Zhao Y. Components of cancer metabolism and therapeutic interventions. Mitochondrion. 2014 Jul;17:50-5. [PUBMED]
- 8Hsu PP, Sabatini DM. Cancer cell metabolism: Warburg and beyond. Cell. 2008 Sep 5;134(5):703-7. [PUBMED]
- 9NCI Staff. "Metabolomics Study Reveals another Energy Source for Cancer Cells ." NIH National Cancer Institute. (2017). [NCI]
- 10Mankoff DA, Bellon JR. Positron-emission tomographic imaging of cancer: glucose metabolism and beyond. Semin Radiat Oncol. 2001 Jan;11(1):16-27. [PUBMED]
- 11Pan JG, Mak TW. Metabolic targeting as an anticancer strategy: dawn of a new era? Sci STKE. 2007 Apr 10;2007(381):pe14. [PUBMED]
- 12 . (2020). Subpopulation targeting of pyruvate dehydrogenase and GLUT1 decouples metabolic heterogeneity during collective cancer cell invasion. Nature Communications, 11(1), 1533. http://doi.org/10.1038/s41467-020-15219-7 (Original work published March 2020) [PUBMED]